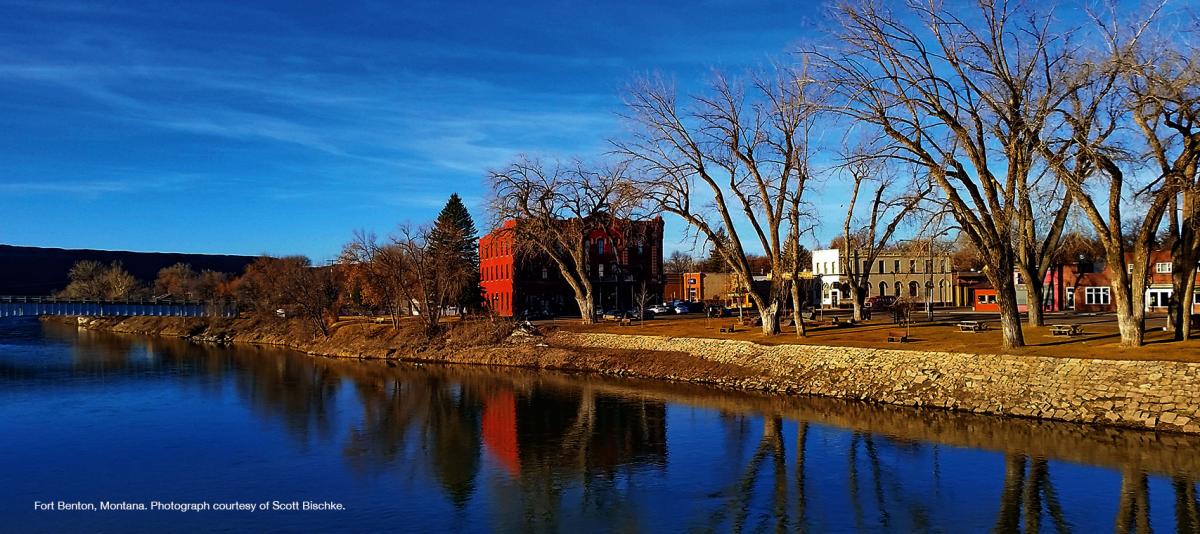
FIGURES
Figure I. Montana’s seven climate divisions.
Figure II. Trends in annual average temperature across each climate division (Figure I) in Montana. The divisions are northwestern (NW), southwestern (SW), north central (NC), central (C), south central (SC), northeastern (NE), and southeastern (SE).
Figure III. The projected increase in annual average daily maximum temperature (°F) for each climate division in Montana for the periods 2049-2069 and 2070-2099 for (A) stabilization (RCP4.5) and (B) business-as-usual (RCP8.5) emission scenarios.
Figure IV. The focal rivers for this assessment, including black outlines of the seven climate divisions (see Water chapter), contributing watersheds (red), river gage locations (green), and the Continental Divide (dotted).
Figure V. Existing forest cover type in Montana (Landfire 2012). Gray boundaries delineate climate divisions (see Figure I).
Figure VI. Factors that drive agricultural decisions in Montana. The size of bubble and arrows qualitatively represents the relative importance of each factor’s influence on agricultural production decisions.
Figure 1-1. Global climate projections from the Intergovernmental Panel on Climate Change, showing temperature and precipitation trends for two different future scenarios, as described in the Climate chapter of this assessment (IPCC 2014a).
Figure 2-1. Changes in important global atmospheric greenhouse gas concentrations from year 0 to 2005 AD (ppm, ppb = parts per million and parts per billion, respectively) (Forster et al. 2007).
Figure 2-2. Montana is the fourth largest state in the nation and provides the headwaters for three major river basins. Two of these, the Columbia and the Missouri, encompass almost 1/3 of the landmass of the conterminous US. The Continental Divide is the line running through the state, and forming the Montana/Idaho border until reaching Wyoming.
Figure 2-3. Montana’s seven climate divisions.
Figure 2-4. Trends in annual average temperature across each climate division (Figure I) in Montana. The divisions are northwestern (NW), southwestern (SW), north central (NC), central (C), south central (SC), northeastern (NE), and southeastern (SE).
Figure 2-5. Typical January-March weather anomalies and atmospheric circulation during El Niño (top) and La Niña (bottom) events. Image courtesy National Weather Service (NWSa undated).
Figure 2-6. (A) Top two images show the average anomaly in Montana’s winter precipitation (left) and temperature (right) during La Niña events. (B) Bottom two images show the average anomaly in Montana’s winter precipitation (left) and temperature (right) during El Niño events. For Montana, El Niño winters are generally drier and warmer; La Niña winters are generally wetter and colder. This analysis was done using data from Livneh et al. (2013) and is based on the study period of 1915-2013.
Figure 2-7. (A) Top two images show the average anomaly in Montana’s winter precipitation (left) and temperature (right) during the cool phase of the Pacific Decadal Oscillation. (B) Bottom two images show the average anomaly in Montana’s winter precipitation (left) and temperature (right) during the warm phase of the Pacific Decadal Oscillation. For Montana, the warm phase of the Pacific Decadal Oscillation is generally associated with warmer and drier winters. Cool phase Pacific Decadal Oscillation winters are generally wetter and colder. This analysis was done using data from Livneh et al. (2013) and is based on the study period of 1915-2013.
Figure 2-8. Example of a simple linear regression model of climate change. This model looks at the historical data of a climate variable (e.g., temperature) and has a best-fit line running through these data. This best-fit line follows the same trend into the future and can be used to project the change of the climate variable in the coming years. Such a model is useful to illustrate modeling principles, but it is too simple to accurately forecast future climate trends.
Figure 2-9. Graphs showing the minimum, maximum, and median temperature increases (°F) projected for each climate division in both stabilization (RCP4.5) and business-as-usual (RCP8.5) emission scenarios. The top row shows mid-century (2040-2069) projections and the bottom row shows end-of-century (2070-2099) projections. The outline of each box is determined by model agreement on the sign of the change (positive or negative). A black outline means there is >=80% model agreement and a red outline means that there is <80% model agreement. In this case, all models indicated the direction of the temperature trend at an agreement of greater than 80%.
Figure 2-10. The projected increase in annual average daily maximum temperature (°F) for each climate division in Montana for the periods 2049-2069 and 2070-2099 for (A) stabilization (RCP4.5) and (B) business-as-usual (RCP8.5) emission scenarios.
Figure 2-11. The projected monthly increase in average temperature (°F) for each climate division in Montana in the mid-century projections (2040-2069) for the (A) stabilization (RCP4.5) and (B) business-as-usual (RCP8.5) emission scenarios.
Figure 2-12. The projected increases in number of days above 90°F (32°C) for each climate division in Montana over two periods 2040-2069 and 2070-2099 for (A) stabilization (RCP4.5) and (B) business-as-usual (RCP8.5) emission scenarios.
Figure 2-13. Graphs showing the increase in the number of days per year above 90°F (32°C) projected for each climate division in both stabilization (RCP4.5) and business-as-usual (RCP8.5) emission scenarios. The top row shows mid-century projections (2040-2069) and the bottom row shows end-of-century projections (2070-2099). The outline of each box is determined by model agreement on the sign of the change (positive or negative). A black outline means there is >=80% model agreement and a red outline means that there is <80% model agreement. In this case, all models indicated the direction of the trend for days above 90°F (32°C) at an agreement of greater than 80%.
Figure 2-14. The projected change in the number of frost-free days for each climate division in Montana over two periods 2040-2069 and 2070-2099 for (A) stabilization (RCP4.5) and (B) business-as-usual (RCP8.5) emission scenarios.
Figure 2-15. Graphs showing the increases in frost-free days/yr projected for each climate division in both stabilization (RCP4.5) and business-as-usual (RCP8.5) emission scenarios. The top row shows mid-century projections (2040-2069) and the bottom row shows end-of-century projections (2070-2099). The outline of each box is determined by model agreement on the sign of the change (positive or negative). A black outline means there is >=80% model agreement and a red outline means that there is <80% model agreement. In this case, all models indicated the direction of the trend of frost-free days at an agreement of greater than 80%.
Figure 2-16. The projected change in annual precipitation (inches) for each climate division in Montana over two periods 2040-2069 and 2070-2099 for (A) stabilization (RCP4.5) and (B) business-as-usual (RCP8.5) emission scenarios.
Figure 2-17. Graphs showing annual precipitation change (in inches) projected for each climate division in both stabilization (RCP4.5) and business-as-usual (RCP8.5) emission scenarios. The top row shows mid-century projections (2040-2069) and the bottom row shows end-of-century projections (2070-2099). The outline of each box is determined by model agreement on the sign of the change (positive or negative). A black outline means there is >=80% model agreement and a red outline means that there is <80% model agreement. In this case, all models indicated the direction of the annual precipitation trend at an agreement of greater than 80%.
Figure 2-18. Graphs showing the minimum, maximum, and median percent changes in annual precipitation projected for each climate division in both stabilization (RCP4.5) and business-as-usual (RCP8.5) emission scenarios. The top row shows mid-century projections (2040-2069) and the bottom row shows end-of-century projections (2070-2099). The outline of each box is determined by model agreement on the sign of the change (positive or negative). A black outline means there is >=80% model agreement and a red outline means that there is <80% model agreement. In this case, all models indicated the direction of the precipitation trend at an agreement of greater than 80%.
Figure 2-19. Graphs showing the interannual variability of precipitation projected for each climate division in both stabilization (RCP4.5) and business-as-usual (RCP8.5) emission scenarios. The top row shows mid-century projections (2040-2069) and the bottom row shows for end-of-century projections (2070-2099). The outline of each box is determined by model agreement on the sign of the change (positive or negative). A black outline means there is >=80% model agreement and a red outline means that there is <80% model agreement.
Figure 2-20. Projected monthly change in average precipitation (inches) for each climate division in Montana in the mid-century projections (2040-2069) for (A) stabilization (RCP4.5) and (B) business-as-usual (RCP8.5) emission scenarios.
Figure 2-21. The projected monthly change in average precipitation (inches) for each climate division in Montana in the end-of-century projections (2070-2099) for (A) stabilization (RCP4.5) and (B) business-as-usual (RCP8.5) emission scenarios.
Figure 2-22. The projected change in the number of consecutive dry days (<0.1 inch [0.3 cm] of precipitation) for each climate division in Montana over two periods 2040-2069 and 2070-2099 for (A) stabilization (RCP4.5) and (B) business-as-usual (RCP8.5) emission scenarios.
Figure 2-23. Graphs showing the number of consecutive dry days in a year projected for each climate division in both stabilization (RCP4.5) and business-as-usual (RCP8.5) emission scenarios. The top row shows mid-century projections (2040-2069) and the bottom row shows end-of-century projections (2070-2099). The outline of each box is determined by model agreement on the sign of the change (positive or negative). A black outline means there is >=80% model agreement and a red outline means that there is <80% model agreement. In the case of consecutive dry days, there was less than 80% agreement across the models for all climate divisions.
Figure 2-24. Graphs showing the increase in the number of wet days/yr projected for each climate division in both stabilization (RCP4.5) and business-as-usual (RCP8.5) emission scenarios. The top row shows projections for mid century (2040-2069) and the bottom row shows projections for end-of-century (2070-2099). The outline of each box is determined by model agreement on the sign of the change (positive or negative). A black outline means there is >=80% model agreement and a red outline indicates <80% model agreement. Model agreement for the trend of wet days each year was greater than 80%, except for the northeastern climate division.
Figure 3-1. Simplified schematic of the water cycle. Artwork by Jenny McCarty.
Figure 3-2. Mean annual precipitation for the years 1981-2010 from Daymet. Daymet is produced by the Oak Ridge National Laboratories from methods originally developed at the University of Montana. The data are derived from elevation and daily observations of precipitation in inches from ground-based meteorological stations. Figure courtesy Montana Climate Office.
Figure 3-3. Statewide average annual flow accumulation as inflows and outflows in millions of acre-feet/yr (1 acre-foot = 1233 m3). Image from the Montana State Water Plan 2015, courtesy of the Montana Department of Natural Resources and Conservation (MT DNRC 2015).
Figure 3-4. Distribution of surface-level (i.e., surficial) and bedrock aquifers across Montana. Images from MT DNRC, Montana State Water Plan 2015 (MT DNRC 2015).
Figure 3-5. The focal rivers for this assessment, including black outlines of the seven climate divisions (see Water chapter), contributing watersheds (red), river gage locations (green), and the Continental Divide (dotted).
Figure 3-6. Streamflow patterns throughout the year for our focal rivers, including the average, 10th percentile, and 90th percentile flow for the long-term periods of record. Flow is in cubic feet per second or CFS (metric unit is m3/s).
Figure 3-7. Trends in April snowpack in the western US, 1955-2016. Red bubbles indicate areas with declining snowpack; blue bubbles indicate areas with increasing snowpack. The diameter of the bubbles is proportional to the percentage change between 1955 and 2016. Figure from Mote and Sharp (2016).
Figure 3-8. Snow water equivalent (SWE) reconstruction for the Northern Rockies based on tree-ring measurements (figure from Pederson et al. 2013a). Z-scores standardize the data to represent the number of standard deviations above or below the long-term average.
Figure 3-9. Normalized April 1 SWE based on Snow Course measurements west and east of the Continental Divide. The upper panel in each column shows data summarized from all Snow Course stations west or east of the Continental Divide. The middle and lower panels show patterns of SWE at high or lower elevations. Black lines represent simple downward trends and are not meant for statistical inference.
Figure 3-10. APRIL 1 SWE projections for three snowmelt-dominated basins in Montana under two scenarios (RCP4.5 and RCP8.5) and two time periods (2040-2069 and 2070-2099). Data are presented as the projected percent change in April 1 SWE between the baseline period 1970-2000 and two future time periods (2040-2069: upper panel; 2070-2099: lower panel). Box and whiskers plots show variation in projections among the different models. These types of plots appear in other graphs below that depict model projections.
The line in the middle of the boxplot represents the median value of all model projections. The bottom and top of the box represent the 25th and 75th percentiles (or first and third quartiles), respectively, of model projections. The upper whisker (line extending from the box) extends from the box to the largest model value no further than 1.5*IQR from the box (where IQR is the inter-quartile range, or distance between the first and third quartiles). The lower whisker extends from the box to the smallest model projection that is no further than 1.5*IQR of the hinge. Few model projections fall beyond the end of the whiskers (i.e., outliers), and these are not shown in the figures.
For explanation of specific confidence levels, refer to Future Projections in Water Chapter.
Figure 3-11. Observed and projected trends demonstrating a general shift toward earlier snowmelt and spring runoff in many regions of the west. Data represent observed and projected shifts in the center of timing of streamflow. Projected trends in center of timing for 2080-2099 are compared to a baseline of 1951-1980 (Stewart et al. 2004).
Figure 3-12. Monthly streamflow projections for each of our focal rivers based on RCP8.5 and time period 2040-2069. Data are presented as the projected percent change in runoff between 2040-2069 and the baseline period of 1970-2000. (Boxplots are explained in the caption of Figure 3-10.) For explanation of specific confidence levels, refer to Future Projections in Water Chapter.
Figure 3-13. Long-term patterns of total annual streamflow in our focal rivers. Each panel shows the annual discharge (gray line) expressed as cubic feet per second or CFS (metric unit is m3/s). The blue and red lines show the percentage deviation above (blue) or below (red) the long-term average for each year. The dark black line represents the 5-year moving average. The red shading represents the most significant periods of hydrologic drought for each focal river.
Figure 3-14. Climate factors associated with naturalized streamflow in four Montana river basins. The size of pie pieces correspond to how strong the particular climate factor influences total annual streamflow. Some of these factors lead to greater flow (positive), while others lead to reduced annual flow (negative). See text for further explanation.
Figure 3-15. Total annual streamflow projections for the focal rivers under RCP4.5 and RCP8.5 for 2040-2069. Data are presented as the projected percent change in runoff between 2040-2069 and the baseline period of 1970-2000. (Boxplots are explained in the caption of Figure 3-10.) For explanation of specific confidence levels, refer to Future Projections in Water Chapter.
Figure 3-16. Montana is divided into two physiographic regions: the intermontane basins of the northern Rocky Mountains, and the northern Great Plains of eastern Montana.
Figure 3-17. There are roughly 200,000 wells (tiny black dots in figure) that provide water for a variety of uses: a) most wells are for domestic and stock use; b) most withdrawals are for irrigation and public water supply.
Figure 3-18. More than 900 wells (black dots) obtain water from the Madison Limestone aquifer near Great Falls. The Madison Limestone is exposed at the surface in the Little Belt Mountains (blue area on map), but is more than 400 ft (120 m) below the surface at Great Falls (MBMGb undated).
Figure 3-19. Between 1995 and 2005, the number of wells drilled into the Madison Limestone aquifer around Great Falls nearly doubled. During the same period, water levels in the aquifer dropped by 30 ft (9 m). However, this was also a dry period, as indicated by the departure from average precipitation plot above. Water levels recovered following several wet years, even though wells continued to be drilled into the aquifer. Location of the hydrograph wells is shown in Figure 3-18.
Figure 3-20. Hydrographs for two wells completed in the same aquifer near the Bitterroot River show very different responses. The well near Hamilton is downgradient from several irrigation canals and irrigated fields; the well near Florence is not located near irrigation. The average monthly water levels show the difference in seasonal response of groundwater levels and highlight the importance of irrigation water as a source of recharge to the shallow aquifers (MBMGb undated).
Figure 3-21. Water levels in the Fox Hills–Hell Creek aquifer near Terry are declining at a rate of about 1 ft/yr (0.3 m/yr) (MBMGb undated).
Figure 3-22. Relative influence of temperature and precipitation on August flows for the focal rivers of this assessment. In general, warmer temperatures have a negative influence on August streamflow, while precipitation has a positive influence on flows. Differences exist among seasons and rivers.
Figure 4-1. Existing land cover in Montana (Landfire 2012). Gray boundaries delineate climate divisions: 1-northwestern, 2-southwestern, 3-north central, 4-central, 5-south central, 6-northeastern, 7-southeastern (see Climate chapter).
Figure 4-2. Percent forest ownership in Montana (adapted from MT DNRC 2010).
Figure 4-3. Existing forest cover type in Montana (Landfire 2012). Gray boundaries delineate climate divisions (see Figure 2-3).
Figure 4-4. Extent and location of historical and recent fires in Montana, 1889-2013. Historical data (1889-1991) are mapped as actual fire boundary polygons as available. Recent data (1992-2013) are mapped as circles approximating burned area. Recent fires too small to be seen by area are mapped as points. Forests are shown in green. Fire data represent primarily forest fires, but may include grassland and other fire types. Brown boundaries delineate climate division. Data and map from Hoff (forthcoming).
Figure 4-5. Fire severity (measured as total carbon stored in aboveground tissues killed by fire) estimated for 2003-2012, a relatively dry decade. Adapted from Berner et al. (forthcoming).
Figure 4-6. Number of fires in Montana, 1970-2015, by month of occurrence (NIFC undated).
Figure 4-7. Recent Montana forest disturbance as visually estimated from aerial surveys in 2000-2015 (USFS 2016). Forests are shown in green. Darker gray background represents area surveyed in 2015; not all areas were surveyed in all years and many pathogens cannot be visually estimated. Brown boundaries delineate climate divisions.
Figure 4-8. Forest disturbance in Montana from 2000-2015 by type of visually surveyed pathogen or insect as percentage of the total area surveyed from USFS (2016) Aerial Detection Survey data.
Figure 4-9. Forested areas (green) at high risk of mortality (red) from combined insect and pathogen attacks from the National Insect and Disease Risk Map (Krist et al. 2014). This map does not consider increased risks from projected climate changes. Areas in red are locations where it is estimated that 25% or more of live trees with a diameter of greater than 1 inch (2.5 cm) are at risk of mortality by 2027 from insects and disease.
Figure 5-1. Mean changes in hail (diameter ≥ 1.0 cm) event days per season from the present (1971–2000) to the future (2041–2070) for spring (March-May) (left) and summer (June-August) (right) based on multiple model simulations. Colored cells indicate mean changes for all model pairings that agree on the direction of change; cells with colored circles indicate mean changes for at least two model pairings (Brimelow et al. 2017).
Figure 5-2. Interactions of natural systems and human interventions guarantee that climate change effects on agriculture, and vice versa, will be neither simple nor trivial.
Figure 5-3. The proportion of total wheat acres planted each year in Montana as winter wheat (USDA-NASS 2015).
Figure 5-4. Acres of corn planted each year in Montana, including that grown for silage (USDA-NASS 2015).
Figure 5-5. Factors that drive agricultural decisions in Montana. The size of bubble and arrows qualitatively represents the relative importance of each factor’s influence on agricultural production decisions.
Figure 5-6. The difference between irrigated and non-irrigated hay production (i.e., irrigated hay production - non-irrigated hay production), which includes grass and alfalfa (USDA-NASS 2015).